Research Projects
Explore our ongoing and completed research projects to understand our expertise and capabilities.
Dynamics of Cancer Development
Cancer starts after initially healthy tissue cells accumulate several specific mutations or other genetic alterations. The dynamics of cancer development is a very complex phenomenon due to multiple involved biochemical and biophysical processes. In our approach, the cancer initiation process is viewed as a set of stochastic transitions between discrete states defined by the different numbers of mutated cells. We evaluate the dynamic processes associated with the cancer initiation using a discrete-state stochastic description of the formation of tumors as a fixation of cancerous mutations in tissues.
Unlocking the Therapeutic Potential of Peptides: A Machine Learning Approach
Harnessing the power of machine learning to uncover the therapeutic potential of antimicrobial peptides (AMPs) offers an exciting avenue in the fight against a spectrum of threats, from antibiotic-resistant bacteria to fungi and antimalarial parasites. By leveraging bioinformatic tools, we’ve extracted an extensive set of physicochemical descriptors of peptides. Our sophisticated approach employs both supervised and unsupervised machine learning methods to manage this high-dimensional data, pinpointing the pivotal physicochemical features that empower peptides to combat bacteria, fungi, and malaria parasites effectively.
Theoretical Description of the Action of Antimicrobial Peptides on Bacteria
The emergence of multidrug-resistant bacteria and limitations in the number of bacterial targets remain among the main challenges in discovering new antibacterial therapies. Antimicrobial peptides (AMPs) are compounds naturally produced during immune responses of living organisms against bacterial infections, making them a strong alternative to conventional antibiotics. We study the activity of antimicrobial peptides, both in combination and alone, against bacteria using first-passage stochastic methods, Monte-Carlo simulations as well as mean-field descriptions.
Development of Morphogen Gradient Formation
The fundamental processes of biological development are governed by multiple signaling molecules that create non-uniform concentration profiles known as morphogen gradients. It is widely believed that the establishment of morphogen gradients is a result of complex processes that involve diffusion and degradation of locally produced signaling molecules. We are developing discrete-state stochastic models for investigating the corresponding reaction-diffusion models.
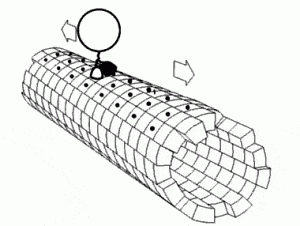
Mechanisms of Motor Protein Transport
A group of catalytic proteins, known as motor proteins, such as kinesins, dyneins, myosins, DNA and RNA polymerases operate in biological cells by consuming energy provided by ATP hydrolysis. They play crucial roles in cell division, cellular transport, muscle contraction and genetic transcription. Current experimental techniques allow measurements of biochemical and mechanical properties of motor proteins with single-molecule precision. However, the main fundamental question related to motor proteins – how the chemical energy is transformed into mechanical motion – is still unanswered. We are developing stochastic models of the motion of motor proteins which take into consideration the biochemical complexity of these processes. Our theoretical methods will be used to describe existing and future experiments on motor protein transport.
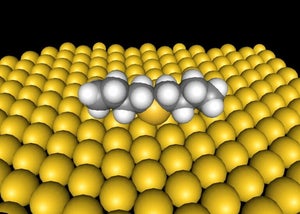
Dynamics of Surface-mounted Thioethers
Recent single-molecule experiments indicated that thioethers (dialkylsulphides) deposited on the gold surface may act as thermally or mechanically activated molecular motors, although the factors affecting the properties of such surface-mounted rotors are not yet clearly understood. In particular, it was found that for the thioethers containing up to six carbon atoms in each chain the rotational energy barriers are almost independent on the alkyl chain length with the only exception of the dimethylsulphide for which the rotation is almost barierless. This observation contradicts the naive assumption that the rotation activation energies should increase with the increase of the molecule size. We use molecular dynamics simulations to study the dynamics of thioethers and other surface-mounted rotors.
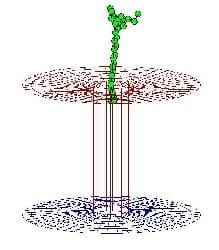
Dynamics of Polymer Translocation across Nanopores
The transfer of DNA, RNA and proteins through cell membranes is fundamental to the understanding of multiple biological processes. The transport of linear polymer molecules across the nanopores is also important in many chemical and industrial processes. However, our theoretical understanding of these complex phenomena is still very limited. Experiments suggest that the dynamics of translocation strongly depends on the size, flexibility, and chemical and electrostatic interactions between the polymers and the pores. We are developing theoretical models which explicitly take into account these properties.
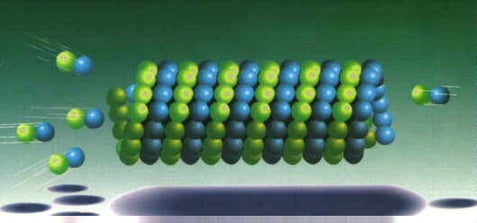
Polymerization/Depolymerization Processes in Microtubule and Actin Filaments
Microtubules and actin filaments are rigid cylindrical biopolymers which are important in cell division, in internal organization of cells and in cell motility. It is known that the growth of microtubules and actin filaments generate forces which determine the biological functioning of these biopolymers. We are developing detailed models of microtubule and actin filaments growth which take into account the complex structure and the lateral interactions of monomers in these biopolymers. We are also interested in explaining the dynamic instability phenomena in microtubules and the coupling between actin filaments growth and cell membrane tension. Our theoretical work is done in collaboration with experimental group of Prof. W. Brownell from Baylor College of Medicine.
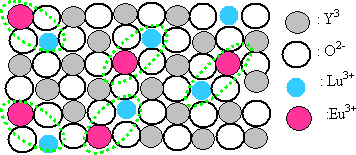
Thermodynamics of Electrolytes
Electrolytes play important roles in science and industry. However, the complete thermodynamic description of these systems is still an open question. Current experimental and theoretical studies are focused on critical properties of ionic fluids where controversial results exist. We are developing Debye-Huckel-based theories for lattice models of electrolytes. Our theories are focusing on the effects of size- and charge asymmetry of charged particles on macroscopic thermodynamic properties. We are checking our theoretical predictions with extensive computer simulations. In this area our collaborator is Prof. Panagiotopoulos from Princeton University.
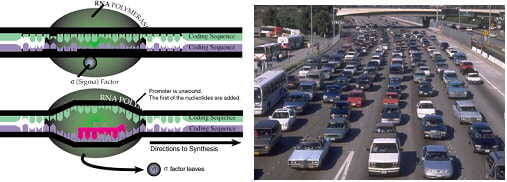
1D Nonequilibrium Multi-Particle Transport
Multi-particle transport phenomena are important for understanding the mechanisms of nonequilirbrium processes in chemistry, physics and biology (such as gel electrophoresis, kinetics of biopolymerization, ion channels, traffic problems, polymer dynamics, surface growth, anomalous conductivity). The complexity of these problems arises from the nonequilibrium nature of these phenomena. We are investigating the effects of detachments/attachments, inhomogeneity, chemical processes and particles size on transport properties of interacting particles. These problems are studied by the means of exact analysis, mean-field theories and Monte Carlo simulations.
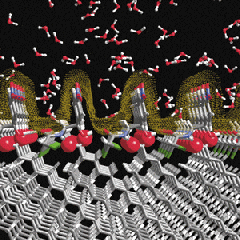
Theory of Biocrystallization Processes
Recently, we started a new project on theoretical modeling of biocrystallization processes, mainly protein crystallization. The range of molecule-molecule interactions in proteins, (in contrast to simple compounds like water, nitrogen, etc.) is much smaller than the size of the molecules. This leads to a complex dynamics of crystallization, which is not well understood theoretically. We are developing simple models of protein crystallization which take into account the intermediate states and phase transitions. Our theoretical efforts are accompanied by experimental work with Prof. P. Vekilov from University of Houston.
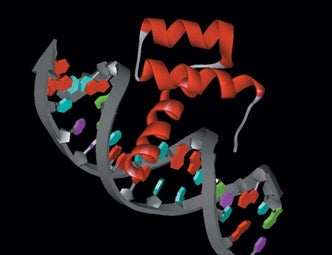
Protein-DNA Interaction
Protein searching and recognizing the targets on DNA was the subject of many experimental and theoretical studies. It is often argued that some proteins are capable of finding their targets 10-100 times faster than predicted by the three-dimensional diffusion rate. However, recent single-molecule experiments showed that the diffusion constants of the protein motion along DNA are usually small. This controversy pushed us to revisit this problem. We are investigating this problem both analytically and computationally to throw some light into the physical-chemical aspects of the target search and recognition. Currently we are performing extensive Monte Carlo simulations.
Create Your Own Website With Webador